I have an impression that there is a large disparity between how the public views museums and how scientists who use museums view them. Presumably there are survey data on public attitudes, but surely the common impression is that museums mainly exist to exhibit cool stuff and educate/entertain the public. Yet, furthermore, I bet that many members of the public don’t really understand the nature of museum collections (how and why they are curated and studied) or what those collections even look like. As a researcher who tends to do heavily specimen-oriented and often museum-based research, I thought I’d take the opportunity to describe my experience at one museum collection recently. This visit was fairly representative of what it’s like, as a scientist, to visit a museum with the purpose of using its collection for research, rather than mingling with the public to oggle the exhibits — although I did a little of that at the end of the day…
Stomach-Churning Rating: 4/10; mostly bones except a jar of preserved critters, but also some funky bone pathologies! Darwin hurls once, totally blowing chunks, but only in text.

Early camel is sitting down on the job at the NHMLA.
About two weeks ago, I had the pleasure to spend a fast-paced day in the Ornithology collection of the Natural History Museum of Los Angeles County (NHMLA or LACM). I arranged the visit (you have to be a credible researcher to get access; luckily I seemed to be that!) via email, took an Uber car to the museum, and was quickly cut loose in the collection. I was hosted by the Collections Manager Kimball Garrett, who is an avid birder (adept at citizen science, too!) and a longtime LA native.
Amongst museum curators and collections managers (there can be a distinction between the two but here I’ll refer to them all as “curators”), there is a wide array of attitudes toward and practices with museum collections, regarding how the curators balance their varied duties of not only making the museum collection accessible to researchers (via behind-scenes studies) and the public (via exhibits and behind-scenes tours etc.), but also curation (maintaining a record of what they have in their collection, adding to it, and keeping the specimen in good condition), research, admin, teaching and other duties.
Most curators I’ve known, like Kimball, are passionate about all of these things, and very generous with their time to help scientists make the most of the collection during their visit, offering hospitality and cutting through the bureaucracy as much as possible to ensure that the science gets done. There are those few curators that aren’t great hosts because they’ve had a bad day or a bad attitude (e.g. obsession with paperwork and finding obstacles to accessing specimens for research; or just not responding to communication), but they are few and far between in my experience.
Regardless, the curator is the critical human being that keeps the wheels of specimen-based museum research rolling, and I am appreciative of how deeply dedicated and efficient most curators are. Indeed, I enjoy meeting and chatting with them because they tend not only to be fun people but also incredibly knowledgeable about their collection, museum, and area of expertise. Sadly, this trip was so time-constrained that I didn’t get much time at all for socializing. I had about five hours to get work done so I plunged on in!
Images, as always, can be clicked to emu-biggen them. Thanks to the NHMLA for access!

My initial look down the halls of the osteology storage. Rolling cabinets (on the right) are a typical sight.

Freezers ahoy! With Batman watching over them.

A jar of bats? Why not? Batman approves.

The curator cleared a space on a table for me to set bones on. Then the anatomizing and photographing began!
On entering a museum collection, one quickly gets a sense of its “personality” and the culture of the museum itself, which emerges from the curator, the collection’s history, and the museum’s priorities. There are fun human touches like the ones in the photos below, interspersed between the stinking carcasses awaiting skeletonization, the crumbling bone specimens on tables that need repair or new ID tags, or the rows upon rows of coffee cups ready to fuel the staff’s labours.

Yet another reason why Darwin kicks ass. And fine curator-humour!

Ironic bird pic posted on the wall.

Below a typical wall-hanging of a bovid skull, an atypical display of a clutch of marshmallow peeps. Contest to see whether the mammalian or pseudo-avian specimens last longest?
The NHMLA’s collection is a world-class one, which I why I chose it as the example for this post. When I entered the collection, I got that staggering sense of awe that I love feeling, to look down the halls of cabinets full of skeletonized specimens of birds and be overwhelmed by the vast scientific resource it represents, and the effort it has taken to create and maintain it. Imagine entering a library in which every book had the librarian’s hand in writing and printing it, and that those books’ contents were largely mysteries to humanity, only some of which you could investigate during your visit. Museum collections exist to fuel generations of scientific inquiry in this way. Their possibilities are endless. And that is why I love visiting them, because every trip is an adventure into the unknown– you do not know what you will find. Like these random encounters I had in the collection’s shelves:

Sectioned moa thigh bones, showing thick walls and spars of trabecular bone criss-crossing the marrow cavities.

My gut reaction was that this is a moa wishbone (furcula)- not often seen! It is definitely not a shoulder girdle (scapulocoracoid), which would be larger and more robust, and have a proper shoulder joint. It could, though, be a small odd rib, I suppose. EDIT: Think again, John! See 1st comment below, and follow-ups. I seem to be totally wrong and the ID of scapulocoracoid is right.

A cigar box makes an excellent improvised container for moa toe bones- why not?

Moa feet: all the moa to love!

May the skull of the magpie goose (Anseranas semipalmata) haunt your nightmares.

Healed shank (tibiotarsus) bone of the same magpie goose as above. It had its own nightmares! No mystery why this guy died: vet staff at the zoo tried to fix a major bone fracture (bracing it with tubes and metal spars), and it had time to heal (see the frothy bone formation) but presumably succumbed to these injuries/infection.

Kiwi (Apteryx australis mantelli) hand, showing feather attachments and remnant of finger(s).
Now that I’m in the collection shelves area, it brings me to this trip and my purpose for it! I wanted to look at some “basal birds” for our ongoing patella (kneecap) evolution project, to check which species (or individuals, such as juveniles/adults) have patellae. Every museum visit as a scientist is fundamentally about testing whether what you think you know about nature is correct or not. We’d published on how the patella evolved in birds, but mysteries remain about which species definitely had a patella or how it develops. Museum collections often have the depth and breath of individual variation and taxonomic coverage to be able to address such mysteries, and every museum collection has different strengths that can test those ideas in different, often surprising, ways. So I ventured off to see what the NHMLA would teach me.

Shelves full of boxes, begging to be opened- but unlike Pandora’s box, they release joyous science!

Boxes of kiwis, oh frabjous day! A nice sample size like this for a “rare” (to Northern hemispherites) bird is a pleasure to see.
Well, in my blitz through this museum collection I didn’t see a single damn patella!
As that kneecap bone is infamously seldom preserved in nice clean museum specimens, this did not surprise me. So I took serendipity by the horns to check some of my ideas about how the limb joints in birds in general develop and evolve. One thing I’ve been educating myself about with my freezer specimens and with museum visits (plus the scientific literature) is how the ends (epiphyses) of the limb bones form in different species of land vertebrates (tetrapods). There are complex patterns linked with evolution, biomechanics and development that still need to be understood.

Left side view of the pelvis of a very mature, HUGE Casuarius casuarius (cassowary). The space between the ilium (upper flat bone) and ischium (elongate bone on middle right side) has begun to be closed by a mineralization of the membrane that spanned those bones in life. A side effect of maturity, most likely. But cool- I’ve never seen this in a ratite bird before, that I can recall.

Hatchling ostrich thigh bones (femora), showing the pitted, un-ossified ends that in life would be occupied by thick cartilage.

A more adult ostrich’s femora, with more ossified ends and thinner cartilages.

Rhea pennata (Darwin’s rhea) femora; right (top) one with a major pathology on the knee end; overgrown bone (osteoarthritis?). Owie!

Also very-unfused knee joints of a Darwin’s rhea hatchling. Cool Y-shape!
In birds, most of the bones don’t have anything that truly could be called an epiphysis– the bone ends are capped with thick cartilage that only gradually becomes bone as the birds get older, and even old-ish birds can still have a lot of cartilage (see photos above)– no “secondary centre” (true epiphysis) of bone mineralization ever forms inside that cartilage. However, there are two curious apparent exceptions to this absence of true epiphyses in avian limbs:
(1) in the knee joint, something like an epiphysis forms on the upper end of the tibia (shank bone) and fuses during growth (shown below). Sometimes that unfused epiphysis is confused with a patella, as our recent paper discussed; in any case, where that “epiphysis” came from in avian evolution is unclear. But also:
(2) in the ankle joint, several bones on both sides (shank and foot) of the joint fuse to the long-bones of the limbs, acting like epiphyses. It is well documented by the fossil record of non-avian and avian dinosaurs that these were the tarsals: at least five different bones (astragalus, calcaneum and distal tarsals) were individual bones for millions of years in various dinosaurs, then these all fused to form the “epiphyses” of the shank and foot, eventually completing this gradual fusion within the bird lineage. Modern birds obliterate the boundaries between these five or more bones as they grow.
These are worthwhile questions to pursue because they show us (1) how odd, little-explored features of the avian skeleton came to be; and (2) potentially more generally why limb bones develop the many ways they do in vertebrates, and how they might develop incorrectly — or heal if damaged.
Images below from the NHMLA collections show how this is the case. Fortunately(?) for me, they supported how I thought the “epiphyses” of avian limbs develop/evolved; there were no big surprises. But I still learned neat details about how this happens in individual species or lineages, especially for the knee joint.

Juvenile kiwi’s shank (tibiotarsus) bones viewed from the top (proximal) ends, showing the bubbly nubbins of bone (very bottom of each bone image; lighter region) that are the “cranial tibial epiphyses” often mistaken for patellae.

Subadult kiwi’s tibiotarsi in same view as above, showing the smooth triangular epiphyses fusing onto the tibiae.

Adult kiwi’s tibiotarsi (sorry, blurry photo) in which all fusion is complete.

Looking down at the top/ankle end of the tarsometatarsal (sole) bones in a hatchling ostrich: the three bones are separate and hollow, where “cartilage cones” would have filled them in. The left and right bones have different amounts of ossification; not unusual in such a young bird.

Ossified tendons (little spurs of long, thin bone) on the soles of the feet (tarsometatarsal bones) of a brush-turkey (Alectura lathami)- seldom described in this unusual galliform bird or its close relatives, and thus nice to see. These would be parts of the toe-flexor tendons. Another nice thing about these two tarsometatarsus specimens is that their fusion is basically complete- each is one single bone unit, as in normal adult birds, rather than five or more.
My visit to the NHMLA bird bone collection was a lot of fun, because I got to do what I love: opening box after box of bone specimens, with bated breath wondering what would be inside. In this case, familiarity was inside, but my knowledge of avian bone development and evolution still improved. I got to look at a lot of ostriches, rheas, cassowaries and kiwis, more than I’d seen in one museum before, and that broadened my sample of young, juvenile and adult animals that I’d seen for these species. Their knees and ankles all grew in grossly similar ways, supporting this assumption in my prior work and building my confidence in published ideas. It’s always good to check such things. Each box opened takes some careful observation and cross-checking against all the facts and ideas swirling around in your head. You take notes, scale photos, measurements, do comparisons between specimens, and just explore; letting your curiosity run unleashed as you assemble knowledge, Tetris-like, in your mind.
And I had a lot of fun because a museum collection visit is like swimming in anatomy. You’re surrounded by more specimens than you could ever fully comprehend. Sometimes you run across an odd specimen whose anatomy tells you something about its life, like pathologies such as the terrible fractured magpie goose leg shown above. Or you see some curatorial touch that makes you chuckle at an apparent inside joke or mutter respect for their careful organization in tending their charges. That feeling of pulling open a museum drawer or box lid and peering inside is like few others in science — there might be disappointment inside (e.g. “Crap, that specimen sucks!”), boredom (“Oh. Another one of these!?”) or the joy of discovery (“Holy *@$£, I’ve never seen that before!”). My first scientific publication (in 1998) came from rummaging through the UCMP museum collections as a grad student and spotting an obscure pelvic bone that turned out to be highly diagnostic for the equally obscure clade of bird-like dinosaurs called alvarezsaurids. I happened to open that drawer with the alvarezsaurid specimen at the right time, shortly after the first ever specimen of that dinosaur had been described in the literature (~1994). Before then, no one could have identified what that bone was!
There is time for hours of quiet introspection during museum collection studies, immersed in this wealth of anatomical resources and isolated in a silent, climate-controlled tomb-like hall. It is relaxing and overwhelming at the same time. Especially in my case with just five hours to survey numerous species, you have to budget your time and think efficiently. It’s a unique challenge to explore a museum collection as a researcher. If you don’t learn something — especially in a good museum collection — you’re doing it wrong. In this time of tight finances and trends to close museums or stow away precious collections, it is important to vocally celebrate what a vast treasure museum collections are, and how the people that maintain them are vital stewards of those treasures.
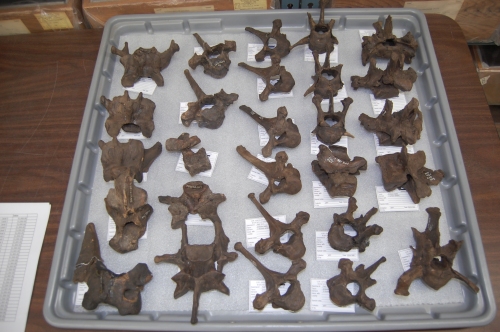
I set the cat amongst the pigeons by also visiting the Page Museum at the La Brea Tar Pits in LA, to study fossil cats– like this American lion (Panthera atrox), code-named “Fluffy”, that we CT scanned during my LA visit– more about that later!
EDIT: I hurried this post off during my free time today, and still feel I didn’t fully capture the deep, complex feelings I have regarding museum collections and the delight I get from studying them. Other freezerinos, please add your thoughts in the Comments below!
Read Full Post »